Variation in solar irradiance and effects on Earth's atmosphere
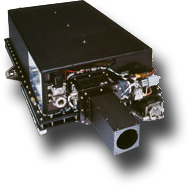
We studied these relationships by analyzing time series of solar irradiance observations made aboard artificial satellites (SOLSTICE experiment) using some spectral techniques to highlight correlations between irradiance variations in the UV spectrum and defining an equivalent of the color index used in astronomy, defined for the FUV (Far UltaViolet) and MUV (Medium UltraViolet) bands, and another widely used index that is the variation of the intensity ratio in the minima and wings of the MgII spectral line, comparing them with stratospheric ozone densities.
Solar flares forecasting

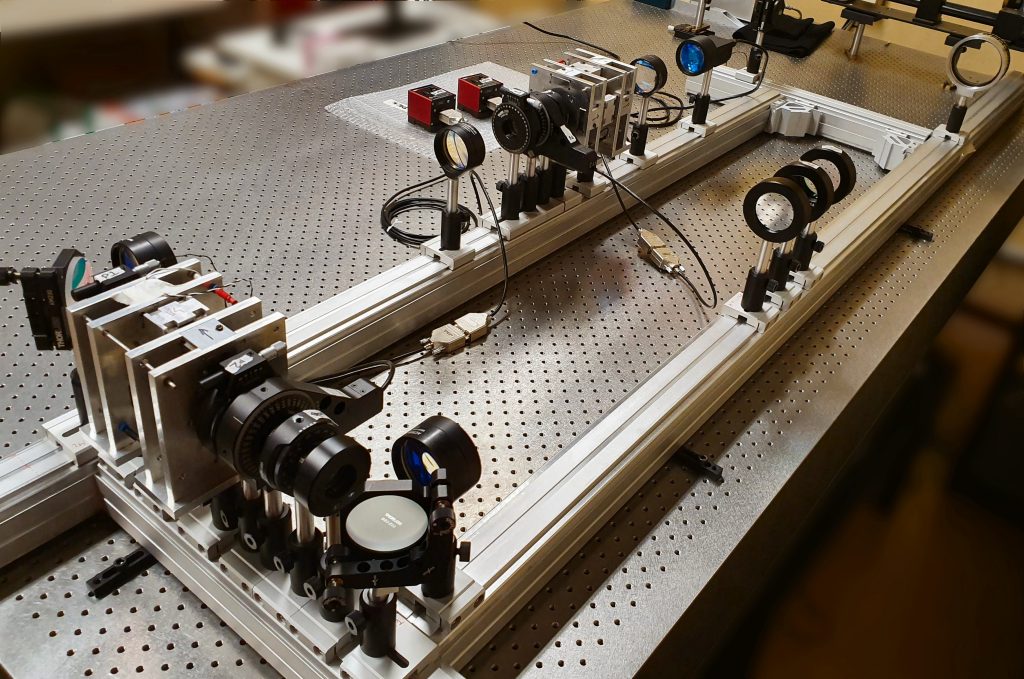
Interaction of coronal mass ejections with the Earth and the circumterrestrial environment
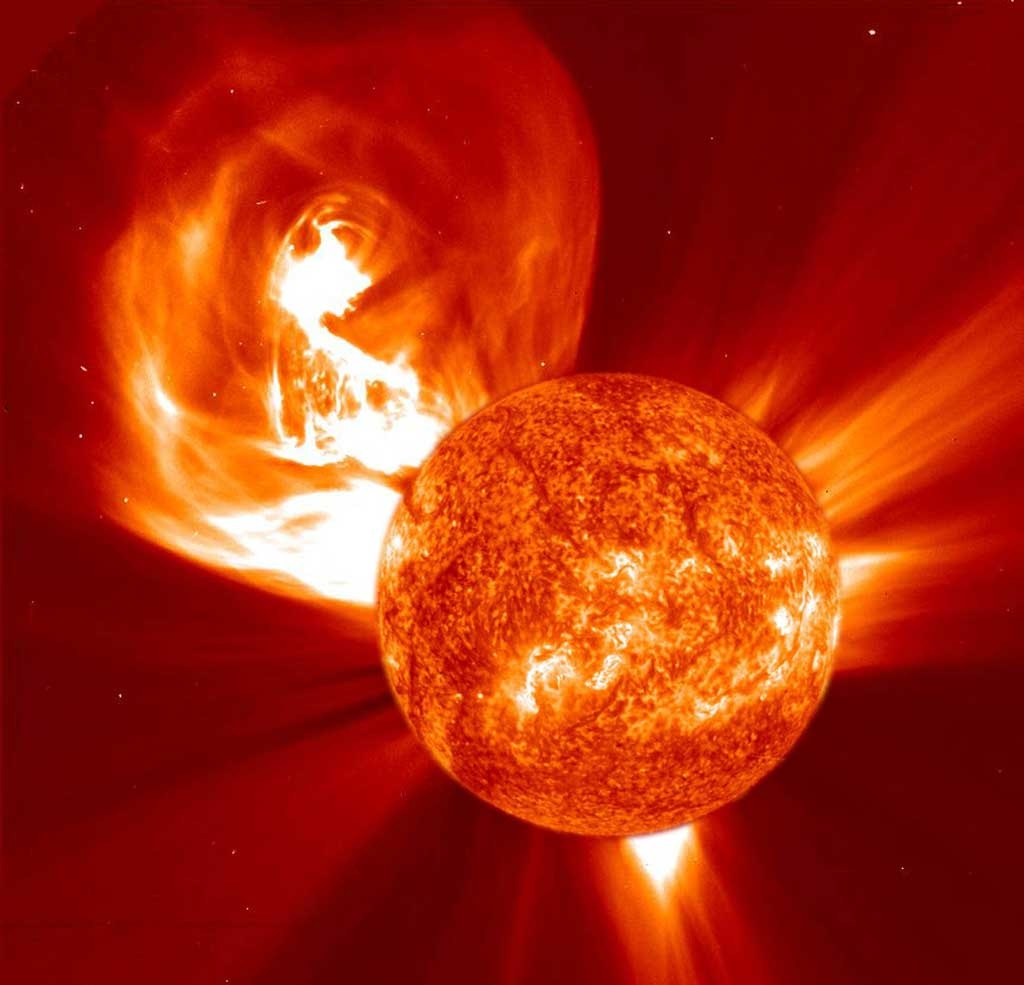
Plasmasphere monitoring

A technique to remotely sense the equatorial plasma mass density in the near-Earth space is based on spectral analysis of Ultra Low Frequency (ULF) magnetic signals recorded by magnetometer arrays on the ground. The technique uses data collected by pairs of magnetometers slightly separated in latitude and approximately aligned along the same magnetic meridian. A latitudinally extended network can monitor the radial dependence of the equatorial plasma mass density along with the longitudinal sector identified by the array. The procedure can be operative on a near real-time basis. EMMA, the European quasi-Meridional Magnetometer Array, is a prototype of this monitoring system established in 2012 in the PLASMON FP7 European project framework. It currently consists of 27 stations and is suitable for determining plasma mass densities for an extended range of L values (1.6-6.2R⊕). 1-sec data are collected and automatically processed every 15 min with an automated procedure to infer the equatorial plasma mass densities.
Solar wind-magnetosphere interaction
The solar wind variability reflects the variability of the solar atmosphere and manifests itself through the solar cycle by different geoeffective structures.
During the declining phase of the solar cycle and near the minimum, high speed streams originate from stable coronal holes. Due to the Sun rotation, they interact periodically (~27 days) with the Earth’smagnetosphere producing a recurrent geomagnetic activity. Near the solar maximum, geomagnetic perturbations are mostly driven by transient structures related to coronal mass ejections.
The interaction of the solar wind structures with the Earth’s magnetosphere produces both global and local geomagnetic field perturbations which occur at different time scales.
ULF waves
Ultra Low Frequency (ULF) fluctuations of the geomagnetic field lines characterize the geomagnetic activity in the frequency range ~1 mHz - 5 Hz. They are observed both in the magnetosphere and on the ground. ULF waves at lower frequencies (1.7-6.7 mHz, Pc5 pulsations on the ground) are believed to be driven by the Kelvin Helmholtz instability along the flanks of the magnetopause and/or by solar wind pressure variations associated to high speed streams. These waves are involved in the acceleration of magnetospheric electrons in the radiation belts.
The main source for ULF waves in the mid-frequency range (10–100 mHz, Pc3 pulsations) is supposed to be represented by upstream waves. They are generated in the Earth's foreshock region by a wave-particle interaction between already existing waves and solar wind protons reflected off the bow shock along the interplanetary magnetic field lines.
ULF higher frequency waves (0.1-5 Hz, magnetospheric EMIC waves, Pc1-2 pulsations on the ground) are generated near the plasmapause by unstable distributions of ring current ions and near the magnetopause by solar wind pressure pulse driven instability. Electromagnetic ion-cyclotron (EMIC) waves are responsible for relativistic electron precipitations at high latitude.